Stars, Planets and Life: Chemistry Controlling the Universe
Mankind has been observing the heavens for millennia. We have peered into the immense depths of space, tuned to the many types of radiation that pervade the Universe. From these observations, we have built up a detailed picture of the structure and evolution of stars, galaxies and of the Universe itself. The Universe is found to be a place rich in atoms, from those primordial light atoms created in the Big Bang to the many types of heavier atom that now populate the periodic table, and which were synthesised by stars in the times since that cataclysmic event. These are the very atoms from which we ourselves are built.
For many years, molecules were thought too fragile to survive the harsh environment of interstellar space. However, some eighty years ago, spectroscopic observations in the visible and near ultraviolet began to show otherwise. At first spectroscopic transitions characteristic of few small discovered. Today some 212 different molecular species have been observed. These range from molecular hydrogen (H2), the most abundant of all molecules, through simple molecules such as water (H2O), methanol (CH3OH) and ethanol (C2H5OH) to complex molecules containing 12 or more atoms. There is even recent evidence for glycine (H2NCH2COOH), the simplest amino acid – one of the constituents of the proteins from which our bodies are assembled.
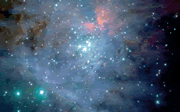
With the observation that such molecules pervade interstellar space came the desire to understand their formation. It was later realised that regions of space that were actively forming stars were also rich in complex molecules (Figure 1, right). This implied an intimate link between the two phenomena. The science of astrochemistry was born from within this environment.
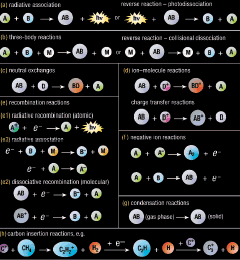
So how are such molecules formed? The very low temperatures and pressures of interstellar space mean that almost all of the chemistry we are familiar with from laboratory studies here on Earth cannot work. Collisions involving two atoms or molecules have too little energy to cross activation barriers and there are too few collisions involving three chemical species to help stabilise newly born molecules in those few reactions without barriers. However, there are a few chemical processes that could operate under the conditions found in the interstellar medium (Figure 2, left). Combining reactions of these types together in complex schemes, astrochemistry can help explain the origin of the observed chemical complexity throughout the Universe.
However, there are still many weaknesses in such models. They often cannot explain the presence of some molecules at all and fail to account for the abundance of others. The most striking example is that of molecular hydrogen. Models using the gas phase chemical processes described in Figure 2 grossly under-estimate the observed concentration of this simple species. Such models are also unable to explain the abundance of other simple molecules eg water. New chemistry is needed to overcome these discrepancies.
In the last few years, astrochemists have come to realise that this new chemistry occurs on the surfaces of interstellar dust grains and that dust grains play three key roles in the chemistry of the interstellar medium:
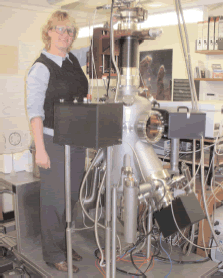
- Grain surfaces provide a means to catalyse the recombination of atoms to form simple molecules. Volatile molecules such as hydrogen produced by this process will be returned to the interstellar medium. Less volatile molecules such as water, ammonia and methane will accumulate as solid ices on the surface of the grains.
- In the coldest regions of the interstellar medium, gases can condense on the surfaces of the grains adding to the chemical richness of the ices produced reactively. Chemical transformations are induced by radiation in this cold environment that converts the relatively simple components of the icy grain mantle into more complex chemical species. Some even speculate that this is the route by which amino acids and other molecules of potential biological significance are synthesised and ultimately delivered to the atmosphere and surface of a young planet seeding it with the precursors to life.
- During star formation, the icy grains are warmed and release this rich inventory of molecules into the gas phase. This is a surprisingly complex process, reflecting both the underlying chemical complexity of the ices and the complexity of their physical structure. Once returned to the gas phase, these molecules may help radiatively cool a collapsing clump of gas as it begins its journey towards being a star, but they may also significantly perturb the gas phase chemistry of the core. Such perturbations may act as markers for different stages in the formation of a star and may give observers a more accurate chemical clock for estimating the age of young stars.
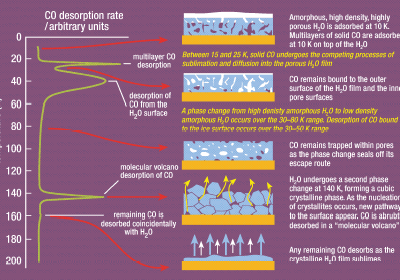
To understand the chemistry and physics of these processes, laboratory astrochemistry has therefore moved into the realm of the surface scientist. An ultrahigh vacuum provides an environment that mimics the denser regions of the interstellar medium associated with star formation (molecular densities of around 106 cm-3 comprising mostly of H2) and we can readily achieve surface temperatures of around 10 K using closed-cycle helium refrigerators. Experimental tools such as infrared spectroscopy and temperature programmed desorption coupled with mass spectrometry then allow us to investigate how strongly, and where, molecules are bound to the surfaces of grains, how rapidly chemical transformations occur within this environment, etc. The complexity of the apparatus involved should not be underestimated (Figure 3, above). However, the measurements that we make are relatively simple and readily reveal the complex behaviour to be found in such low temperature environments (Figure 4, left).
The application of surface science in laboratory astrochemistry is a new and exciting area of scientific research. It has already made some significant contributions to our understanding of the chemical evolution of the interstellar medium and may help answer one of the greatest mysteries of all – how the atomic ashes of previous generations of stars are incorporated into increasingly complex molecules and ultimately into the molecules of life.
For Further Information:
The UCL Centre for Cosmic Chemistry and Physics combines experimental and theoretical studies of gas-grain processes relevant to astrochemistry. It has acted as a focus for both activities in UCL and on a wider scale and couples activity in UCL Physical Chemistry with the UCL Astrophysics Group.
The Astrochemistry Group at Heriot-Watt University is active in experimental studies of surface physics and chemistry relevant to the understanding the gas-grain interaction.
Home page of the Nottingham Astronomy Research Forum describes the activities in astronomy and astrochemistry on going at the University of Nottingham.
The Interdisciplinary Centre for Astrobiology at the Open University is an internationally-known centre combining activities in a wide range of topics in astrochemistry and astrobiology.
The SUPA Astrochemistry Group at Strathclyde has an active programme combining observation and experiment.